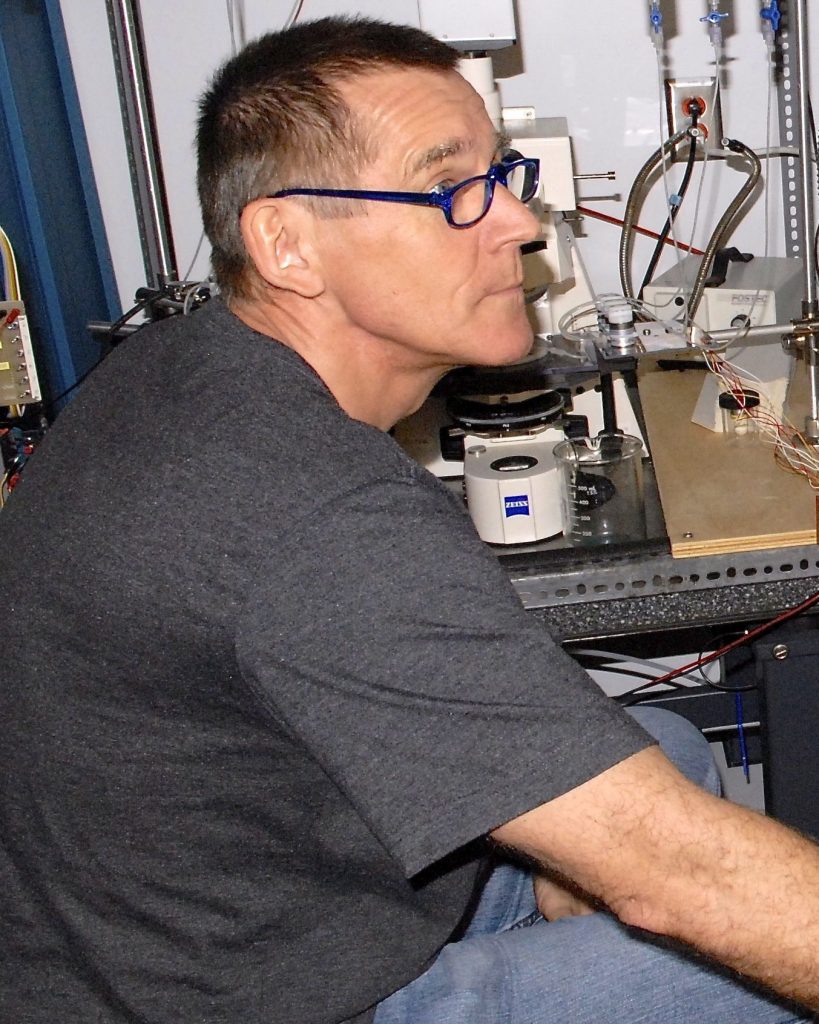
Georgia State University
Petit Science Center
PO Box 5030
Atlanta, GA 30302-5030
mschmidt@gsu.edu
Full Profile
I have been working on various aspects of arthropod neurobiology for more than 30 years. Within this area, the focus is chemoreception of decapod crustaceans which comprise shrimp, spiny lobsters, clawed lobsters, crayfish, true crabs and hermit crabs.
In various species I have studied the physiology of receptor neurons, their projection to processing areas in the central nervous system in particular the brain, and the functional organization of brain pathways processing chemosensory information.
The deeper question driving my research is why chemoreception in crustaceans is differentiated into two morphologically distinct ‘chemical senses’. One of these chemical senses is olfaction (the sense of smell) which is mediated by specialized chemosensory hairs (aesthetasc sensilla) on the first pair of antennae called antennules and a dedicated olfactory pathway in the brain. The other chemical sense is ‘distributed chemoreception’ which is constituted by numerous other chemosensory sensilla that cover the body and all appendages and the associated regions of the ventral nerve cord and brain to which these sensilla send their input. My research question originates from the fact that in contrast to land-living animals where the two main chemical senses are specialized to detect physically different molecules (olfaction detects volatiles whereas taste detects water-soluble molecules) crustaceans are aquatic animals and therefore are exposed to water-soluble molecules only. Since aquatic animals predate terrestrial ones, the evolutionary origin of olfaction can have nothing to do with a specialization for detecting volatiles (which is a later adaptation to terrestrial life) and thus there must be different reason that caused the evolution of the sense of smell. Contributing to elucidate this ‘true’ reason for the evolutionary origin of olfaction is the aim of my work.
My recent work in Chuck Derby’s laboratory, which I joined in 2002, comprises three distinct but interconnected projects:
(1) Functional characterization of spiny lobster chemoreceptor neurons with Ca2+ imaging.
Ca2+ imaging is a method to simultaneously record the activity of several neurons by loading them with a fluorescent Ca2+ indicator dye and then monitoring changes in fluorescence by taking images in rapid succession. We developed a setup that allows performing Ca2+ imaging on chemoreceptor neurons in slice preparations of spiny lobster appendages while focally stimulating sensilla with water-soluble chemicals. In the first, recently finished experiment, we determined with pharmacological treatments that changes in Ca2+ fluorescence in olfactory receptor neurons of aesthetasc sensilla indeed reflect their spiking activity in response to chemical stimulation or occurring as spontaneous bursts. Aim of future experiments is to compare the response properties – sensitivity, tuning to single compounds, spontaneous bursting activity – of olfactory receptor neurons and the chemoreceptor neurons of other sensilla that belong to ‘distributed chemoreception’.

Figure 1: Ca2+ imaging of spiny lobster olfactory receptor neurons (ORNs). Left: fluorescent images of ORNs loaded with a Ca2+ indicator dye before stimulation (A) and at the peak of the response to a chemical stimulus (B). Right: Responses of 4 ORNs highlighted in the images.
(2) Function of antennule grooming and tegumental glands associated with aesthetascs.
All marine decapod crustaceans extensively groom their antennules by pulling them through dense pads of grooming setae on their mouthparts. Clearly, this behavior is directed at the aesthetascs located on the antennules and its overt function is to keep them free of biofouling by the numerous microbes and propagules living in seawater. The cleanliness of the aesthetascs achieved by antennule grooming has generally been attributed to a mechanical scrubbing action of the grooming setae, but according to our current results a sophisticated chemosensory feedback mechanism makes an important contribution.
With behavioral experiments and selective sensilla ablations we identified one component of this mechanism: chemosensory sensilla called asymmetric setae that are associated with the aesthetascs and activate antennular grooming upon stimulation with L-glutamate and some other amino acids among them glycine and L-alanine. Immunocytochemical and electron microscopical analyses of the antennule led to our identification of another component: numerous tegumental glands that have their openings at the base of the aesthetascs and release their secretion upon antennular grooming. The third component is a layer of extracellular material on the cuticular surface of the aesthetascs that was found in a previous electron microscopical analysis (Grünert and Ache, Cell Tissue Res 251:95-103, 1988). The forth component is the previous finding that diverse amino acids – among them L-glutamate, glycine, and L-alanine leak from the aesthetascs into the surrounding seawater (Trapido-Rosenthal et al., Biol Bull 179:374-382, 1990). Combining these components we hypothesize that the surface layer of the aesthetascs minimizes the leakage of amino acids and that it consists of the secretions of the tegumental glands which are distributed over the aesthetasc by antennular grooming. Breach of the surface layer would lead to an increase of amino acid leakage that is detected by the asymmetric setae and through the elicitation of antennular grooming would be ‘repaired’ by the reapplication of gland secretions. According to this scenario, cleanliness of the aesthetascs is a ‘by-product’ of antennular grooming while keeping the aesthetasc surface layer intact seems to be its main function! Aim of future experiments is to elucidate with Ca2+ imaging what role the surface layer has in the normal functioning of the aesthetascs.

Figure 2: (A) Spiny lobster grooming its antennule. (B) Clean, normally groomed antennule with aesthetascs and associated asymmetric setae (AS). (C) Schematic drawing of aesthetascs (AE), their olfactory receptor neurons (ORN) and the associated tegumental glands (ATG). (D) Ablation experiment showing that the elicitation of antennular grooming by stimulation with L-glutamate is mediated by asymmetric setae and not aesthetascs.
(3) Cellular basis of adult neurogenesis in the olfactory midbrain of decapod crustaceans.
Within the past 20 years it has been firmly established that in two regions of the brain of mammals new neurons are continuously generated throughout adult life: one region is the dentate gyrus of the hippocampus, the other one is the olfactory bulb. In a parallel line of research over about the same time period I established that adult neurogenesis also persists in two brain regions of decapod crustaceans, and intriguingly one of them is the olfactory midbrain, the equivalent of the mammalian olfactory bulb. This line of research started in 1997 with the demonstration that continuous adult neurogenesis occurs in the olfactory midbrain of shore crabs and leads to a doubling of olfactory lobe projection neurons over the crab’s life span. Since then I have used a combination of immunocytochemistry, confocal microscopy and electron microscopy to study the neuronal maturation of newly generated cells and their developmental origin in 3 model species: shore crabs, crayfish, and spiny lobsters. One early result was that adult neurogenesis in the olfactory midbrain is originating from small proliferation zones located deep within each of the neuronal soma clusters (2 or 4 depending on the species) in which new neurons are generated. Later I could show that each proliferation zone is associated with one large ‘adult neuroblast’ embedded in a dense ‘clump’ of small, bipolar cells. Likely the adult neuroblast is a continuously self-renewing neural stem cell and the clump of cells its neurogenic stem cell niche. A current electron microscopical analysis revealed that the bipolar niche-forming cells in shore crabs and crayfish have characteristic features of epithelial cells and secrete cuticular material into a central extracellular space – in stalk parallel to imaginal discs of insects. Future experiments aim at identifying key molecular components of the stem cell niche and at elucidating their function in the maintenance of the adult neuroblast and the regulation of its proliferative activity.

Figure 3: (A) Proliferation zone (PZ) in the medial soma cluster (MC) of the spiny lobster brain. Mature neurons labeled with antibodies against the neuropeptides FMRFamide (FMRF) and substance P (SP). Scale bar = 100 µm. (B) Newly generated neuron in the medial soma cluster of the spiny lobster double labeled by an antibody against the S-phase marker BrdU (injected 6 months prior to fixation of brain) and an antibody against substance P (SP) labeling mature neurons. The neuron has a primary neurite (arrowheads) indicative of a mature neuronal phenotype. Scale bar = 10 µm. (C) Neurogenic stem cell niche (NN) in the lateral soma cluster of the spiny lobster. A large BrdU-positive adult neuroblast (aNB) is located at the apex of the neurogenic niche. Duct (D) leading to the proliferation zone is stained with an antibody against phosphorylated Histone H3 (pH3). Scale bar = 10 µm. (D) Neurogenic stem cell niche (NN) in the lateral soma cluster of the spiny lobster labeled by an antibody against Gs/olf and a lectin (ACL). The neurogenic niche is connected to the proliferation zone (PZ) by a duct (D). Scale bar = 100 µm.